The infinite monkey theorem says if you have an infinite number of monkeys randomly pressing keys on typewriters for an infinite amount of time, they will almost surely type out the complete works of William Shakespeare, or any other text, by sheer chance. The idea relies on the concepts of infinity and randomness. Given infinite time and random inputs, every possible text will eventually be produced.

While mathematically valid, the premise highlights the impracticality of relying solely on randomness for complex outcomes -- especially complex biological organisms. Rather, the proposed Template Hypothesis suggests that guided mechanisms, in the form of structural carbon templates called fullerenes, play crucial roles in the early development of biological complexity and functionality, bridging the gap between random events and organized systems.

The Template Hypothesis: Carbon fullerene molecules served as templates or scaffolds for the organization and assembly of prebiotic molecules, facilitating the formation of the first primitive biological structures.
Just as the infinite monkey theorem illustrates the improbability of achieving complex texts purely through random typing, relying solely on random genetic mutations to achieve the complexity observed in biological organisms is similarly improbable. The sheer number of possible mutations and the time required for beneficial mutations to occur and be selected for is immense, making the process inefficient and improbable.
By introducing guided mechanisms, the Template Hypothesis bridges the gap between randomness and the highly organized complexity observed in biological systems. This makes the process of evolving complex traits and organisms more feasible and efficient, akin to having a structured guide rather than relying on the improbable success of random key presses.
Carbon Fullerenes
Fullerenes are a class of carbon molecules with unique cage-like structures. This includes spherical forms (see below) like C60 (made of 60 carbon atoms), C70, C80, all the way up to C180.

Fullerenes can also come in the form of various nanotubes. The images below depict a multi-walled carbon nanotube (MWCNT) and a single-walled carbon nanotube (SWCNT) respectively. MWCNTs are cylindrical structures made of multiple layers of graphene (sheets of carbon atoms arranged in a hexagonal pattern) rolled into concentric tubes. Richard Smalley, co-discoverer of Carbon 60 (C60), said this of them:
These nanotubes are so beautiful that they must be useful for something.




Contrary to the common misconception that fullerenes are solely lab-made, they are ubiquitous both on Earth and in space.
Fullerenes form naturally in carbon-rich environments such as those found in dying stars, interstellar space, and even on Earth. Astronomical observations and analyses of meteorites have shown that fullerenes are present in space, suggesting they could have been delivered to early Earth via meteorites and comets.
Their discovery in 1985 opened new avenues in materials science, electronics, energy storage, and medicine, highlighting their versatile and impactful nature. Not only are they incredibly strong and lightweight due to the powerful carbon-carbon bonds in the graphene layers, they are also known for their electrical conductivity and thermal properties, making them significant in various scientific and technological applications. But the Template Hypothesis suggests there might be more to this story than just creating more efficient solar panels.
Fullerenes as Prebiotic Templates
As we will see in the studies below, the diverse properties of fullerenes align well with the key requirements for a prebiotic template. Their ability to encapsulate and concentrate biomolecules, catalyze important reactions, form stable complexes with key compounds, contribute to the emergence of higher-order structures, and participate in primitive metabolic processes all suggest that they could have been important players in the transition from prebiotic chemistry to early life.
The geodesic structure of fullerenes may have imposed constraints on the evolution of self-replicating systems. These constraints could have influenced the possible pathways and outcomes of evolution, shaping the diversity and complexity of life as we know it. In fact, these geometric constraints may have guided the evolution of metabolic pathways, genetic systems, and other fundamental aspects of life.
Moreover, the capacity for fullerenes to act as multifunctional platforms in prebiotic environments further strengthen the case for their involvement in life's emergence. The variety of possible fullerene-biomolecule interactions and the potential for the emergence of novel structures and functions hint at the evolutionary potential that fullerenes could have provided in prebiotic systems.
While it is true that the Template Hypothesis still requires further experimental validation and integration with existing theories in the field, I believe the evidence presented in the following studies provides a strong foundation for its plausibility. The coherence and complementarity of these findings suggest that the Template Hypothesis merits serious consideration and further investigation.
The Studies
To recap, fullerenes are composed entirely of carbon atoms arranged in a highly stable and repetitive geometric pattern. The carbon-carbon bonds in fullerenes are exceptionally strong, making these molecules chemically stable and resistant to degradation. This stability could have provided a consistent and durable scaffold for early biomolecules to interact whether on Earth or out in space. (e.g. Scientists have discovered amino acids inside of meteorites, right next to fullerenes.)
Studies have shown that fullerenes can encapsulate various biomolecules, such as amino acids, nucleic acids, and even small proteins (e.g., Bosi et al., 2003). This encapsulation could have provided a protective environment for these biomolecules, shielding them from harsh prebiotic conditions and enabling their accumulation and interaction. Also, the larger spherical fullerenes, like C180 and C240, can act as molecular sieves, selectively trapping and concentrating smaller molecules based on their size and shape (Shinohara, 2000).
Experimental studies have also shown that fullerenes can form stable inclusion compounds with various amino acids, such as glycine, alanine, and proline (Cataldo & Iglesias-Groth, 2010). These inclusion compounds could have served as primitive peptide-like structures, potentially contributing to the emergence of early proteins.
Research has shown that fullerenes can interact with lipid membranes and enhance their stability (Wong et al., 2008). In the context of the origin of life, this property could have been important for the formation and stabilization of primitive cell-like structures, such as protobionts or lipid vesicles.
The surface of fullerenes can be chemically modified or functionalized with various groups, such as hydroxyl, carboxyl, or amine groups (Hirsch & Brettreich, 2005). This surface functionalization can alter the properties and reactivity of fullerenes, potentially making them more suited for specific catalytic or templating roles in prebiotic chemistry.
A study by Becker et al. (2000) demonstrated that fullerene C60 can catalyze the formation of sugars from formaldehyde under prebiotic conditions. This finding suggests that fullerenes could have been involved in the synthesis of carbohydrates, essential for energy storage and structural components in biological systems.
Fullerenes can also chelate and form stable complexes with various metal ions, such as Fe, Ni, and Co (Charpin et al., 1991). These metal-fullerene complexes could have served as primitive catalytic centers, resembling the metal cofactors found in modern enzymes.
Fullerenes are capable of undergoing reversible reduction and oxidation reactions, accepting or donating electrons (Reed & Bolskar, 2000). This redox capability could have been important for the emergence of primitive metabolic pathways and energy transduction systems in early life.
Porphyrins are important biological molecules involved in processes such as photosynthesis and oxygen transport. As it happens, studies have shown that fullerenes can form stable supramolecular complexes with porphyrins (e.g., Boyd & Reed, 2005), which could have implications for the emergence of light-harvesting and energy-transducing systems in early life.
Experimental studies have demonstrated that fullerenes can bind to nucleic acids, such as DNA and RNA, and stabilize their structures (e.g., Nakamura & Isobe, 2003). This stabilization could have been crucial in the early stages of life, helping to preserve genetic information and facilitate the replication and evolution of nucleic acids.
Finally, the surfaces of fullerenes have properties that allow organic molecules to adhere to them. This adhesion is facilitated by various forces, including van der Waals interactions, π-π stacking, and hydrophobic interactions. When biomolecules, such as nucleotides, amino acids, or peptides, adhered to the surfaces of fullerenes, they align and orient in a specific manner.
The curved surface of fullerenes can provide a unique templating effect, guiding the assembly of biomolecules into specific structures or patterns. This templating effect has been observed in various studies, such as the formation of peptide nanotubes on the surface of C60 fullerenes (Rozhin et al., 2021).
In fact, according to a study published in 2012, fullerenes may have influenced the initial stages of ribosome assembly and function, contributing to the efficiency and accuracy of protein synthesis.
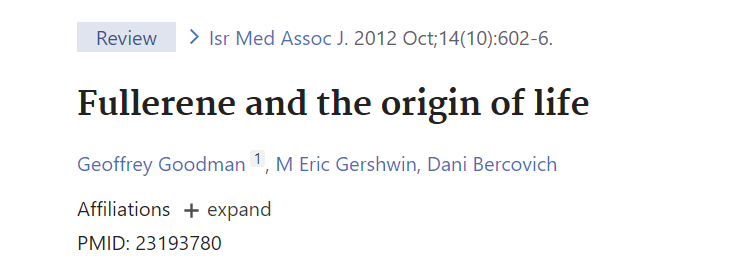
The conclusion of this study: These robust carbon molecules could have acted as early biological templates, facilitating the assembly of small molecules into more complex structures. This process may have initiated evolution, leading to diverse molecular lines that responded to different environments, such as the formation of primordial ribosomes. This parallel evolution explains the integration of DNA, RNA, and ribosomal structures, supporting the idea that life is not unique to Earth and opening new avenues for biotechnology and genetic engineering.
In short, the confined, symmetrical spaces within fullerenes, especially in multi-walled carbon nanotubes (MWCNTs) or natural (12-around-1) Carbon 60 clusters provided a protective environment that stabilized intermediate forms of biomolecules, preventing them from breaking apart or reacting unfavorably.
When considering these studies together, several insights emerge:
Fullerenes as multifunctional platforms: The studies highlight the versatility of fullerenes, showing that they can interact with various biomolecules (amino acids, nucleic acids, lipids) and perform multiple functions (encapsulation, catalysis, stabilization, electron transfer). This suggests that fullerenes could have served as multifunctional platforms in prebiotic chemistry, facilitating diverse processes essential for the emergence of life.
Synergistic effects: The combined effects of fullerenes' properties could have created synergistic advantages in prebiotic environments. For example, the encapsulation and concentration of biomolecules within fullerene cages, coupled with the catalytic properties of functionalized fullerene surfaces, could have enhanced the efficiency and selectivity of prebiotic reactions.
Emergence of higher-order structures: The ability of fullerenes to form stable complexes with biomolecules (e.g., inclusion compounds with amino acids, supramolecular assemblies with porphyrins) suggests that they could have played a role in the emergence of higher-order structures. These complexes could have served as precursors to more advanced functional units, such as primitive enzymes or light-harvesting systems.
Prebiotic compartmentalization: The interaction of fullerenes with lipid membranes and their ability to stabilize these structures suggest a potential role in the formation and maintenance of primitive compartments. Such compartmentalization is considered crucial for the emergence of life, as it allows for the concentration and protection of biomolecules and the creation of distinct chemical environments.
Primitive metabolic processes: The redox properties of fullerenes and their ability to participate in electron transfer reactions hint at their potential involvement in early metabolic processes. Fullerene-mediated redox reactions could have been a source of energy and a driver of chemical transformations in prebiotic systems.
Evolutionary potential: The diverse properties of fullerenes and their ability to interact with various biomolecules suggest that they could have provided a rich playground for prebiotic chemistry. The variety of possible fullerene-biomolecule interactions and the potential for the emergence of novel structures and functions could have increased the evolutionary potential of prebiotic systems.
Bridging the gap between prebiotic chemistry and early life: The studies collectively suggest that fullerenes could have acted as a bridge between the simple prebiotic building blocks and the more complex structures and processes associated with early life. By facilitating the formation, stabilization, and interaction of key biomolecules, fullerenes could have played a crucial role in the transition from prebiotic chemistry to the first living systems.

Therefore, the Template Hypothesis does NOT contradict the prevailing view. In fact, it's complementary.
The prevailing view (abiotic synthesis) is that simple organic molecules, such as amino acids and nucleotides, formed through prebiotic chemical reactions driven by energy sources like UV radiation, lightning, and volcanic activity on early Earth. TTH, rather than contradicting the prevailing view, is complementary. It suggests that fullerenes present in the environment provided catalytic surfaces that facilitated the formation of these organic molecules into more complex forms.
Some studies have investigated the potential of fullerenes to catalyze the formation of amino acids and peptides. For example, a study by Becker et al. (2000) showed that fullerene C60 can catalyze the formation of dipeptides from amino acids under UV irradiation. Research by Ojima et al. (2009) demonstrated that fullerene derivatives can catalyze the polymerization of amino acids, suggesting a potential role in the formation of primitive proteins.
Did We Come From Mud?
The prevailing view also says that specific prebiotic environments might have provided the right conditions for the spontaneous formation and stabilization of RNA strands. Laboratory experiments have shown that nucleotides (basic building blocks of DNA/RNA) can spontaneously polymerize to form RNA strands under certain conditions, suggesting that RNA could form naturally on early Earth. But RNA molecules do not spontaneously form long RNA strands without specific conditions and catalysts.
It's well established that certain inorganic materials played a critical role in aligning and activating nucleotides to facilitate their polymerization into RNA strands. For instance, clay minerals, such as montmorillonite, have been shown to catalyze the polymerization of nucleotides. These minerals provide a surface that aligns the nucleotides and activates them, promoting the formation of phosphodiester bonds necessary for RNA strand formation.
Yet, while clay minerals like montmorillonite are effective at catalyzing nucleotide polymerization, there are several limitations that prevent this mechanism from being entirely conclusive for the formation of RNA strands in prebiotic conditions. For example, RNA molecules, once formed, need to be stable enough to avoid degradation. The catalytic environment provided by clay minerals may not adequately protect nascent RNA strands from hydrolysis and other degradation processes.
A research study by Gournis and colleagues, published in 2017, looked at how fullerenes (specifically, C60) interact with a type of clay mineral called smectite. They found that C60 molecules can insert themselves into the spaces between the layers of the smectite clay. This interaction between the fullerenes and the clay might improve the clay's ability to speed up chemical reactions, which could have implications for understanding how complex molecules formed in the early stages of life on Earth.
In summary, the TTH posits:
Fullerenes, including CNTs, offer stable, uniform, and high-surface-area environments that can enhance the catalytic activity for nucleotide polymerization.
The unique electronic properties of fullerenes can provide additional catalytic support that might not be present with clay minerals alone.
Fullerenes can create microenvironments that protect nascent biomolecules from degradation, increasing their stability and likelihood of forming longer, functional molecules.
The combination of clay minerals and fullerenes could create a synergistic effect, where the strengths of both materials are leveraged. Fullerenes can provide additional catalytic sites and stabilize nascent intermediates, complementing the alignment and activation provided by clay minerals.
The presence of both clay minerals and fullerenes in different prebiotic environments increases the likelihood that RNA formation could occur in a variety of settings, not limited to specific conditions where clay minerals are prevalent.
Conclusion
The fullerene Template Hypothesis suggests that fullerenes played a crucial role in the early stages of molecular evolution by providing catalytic surfaces and structured environments that guided the assembly and folding of biomolecules.
Research in nanotechnology often looks to biological systems for inspiration. If we consider that fullerenes might have been instrumental in the rise of life, it opens up further possibilities for their use in biomedical applications, such as drug delivery, tissue engineering, or enhancing cellular structures.
By bridging the gap between nanotechnology and biology, this hypothesis provides a new framework for understanding how life could have emerged from the simple to the complex. Future research in this area could uncover more about the ancient processes that gave rise to life on Earth and possibly, elsewhere in the universe.
I go more into this idea in my book C60 Science: The God Molecule Hypothesis. Members get a free digital copy of V2 from our library upon release. The first 10 VIP members who collect this post will receive a physical V2 copy shipped to their doorsteps, 100% FREE. The first 10 FREE (non-VIP) subscribers will get access to the digital copy.
*You must be a VIP member to get the physical copy. With a VIP membership, you get access to exclusive content, our monthly investment newsletter, "The Synthesis," and much more.
Addendum: Superradiance of Consciousness
The ORCH-OR theory, short for Orchestrated Objective Reduction, proposes a connection between quantum mechanics and consciousness. Developed by physicist Sir Roger Penrose and anesthesiologist Stuart Hameroff, this theory suggests that quantum processes within the brain's microtubules are fundamental to the emergence of consciousness.
Microtubules are tiny, tube-like structures inside cells that provide support and shape. They are part of the cell's skeleton, known as the cytoskeleton, and play key roles in cell division, movement, and transporting materials within the cell. Think of them as the cell's internal highways, helping to move cargo and organize the cell's components.
Many scientists have disregarded ORCH-OR, viewing it as speculative and lacking empirical support. Critics have long argued that the brain's warm, wet environment is unsuitable for maintaining quantum coherence necessary for ORCH-OR. But the tune is slowly beginning to change in the favor of Penrose and Hameroff. More and more, the prevailing materialist views on consciousness are sounding less like science and a lot more like whistling past the graveyard.
A new study, hot off the presses, supports the idea that the brain uses quantum effects in microtubules, potentially linked to consciousness. The paper shows that microtubules, integral to cell structure and function, exhibit quantum effects such as superradiance.

This reveals that quantum coherence may in fact survive in the warm, noisy environment of the brain, challenging the view that quantum phenomena are too delicate for biological systems. By showing that large networks of tryptophan residues in microtubules can exhibit collective quantum optical behaviors, the study suggests that such effects might play a role in neural processes after all, potentially linking to consciousness as hypothesized by Penrose and Hameroff.

Simplified explanation: shining UV light on tryptophan in biological systems makes them glow brighter due to teamwork among microtubules, and this effect remains strong even with some warm and wet disorder.

Microtubules are made of two types of tubulin dimers (α- and β-tubulin) depicted as blue and purple in the example below. These tubulin dimers are globular (spherical) proteins that polymerize to form long, straight chains called protofilaments. To create the hollow cylindrical structure of microtubules, 13 (12-around-1) of these protofilament chains align side-by-side.

The Template Hypothesis posits that advanced biological structures like microtubules and ribosomes were originally formed with the assistance of prebiotic templates. These templates could have been inorganic surfaces or pre-existing carbon frameworks, such as C60 fullerenes and multi-walled carbon nanotubes (MWCNTs), which provided a stable environment for the assembly of complex biological molecules. In short, the structural and functional sophistication of these cellular components were guided by this primitive scaffolding, aiding the transition from simple molecules to the complex machinery of life, accelerating their expansion to self-sufficient replication. Furthermore, that's precisely where microtubules received their quantum properties, including superradiance.
Metabolic Networks as Geometric Entities
In a paper titled "Uncovering the hidden geometry behind metabolic networks", Serrano et al. provide compelling evidence that the complex structure and function of metabolic networks can be elegantly explained through a hidden circular geometry. The positions of metabolites and reactions on this circle, based on their angles, show how they are related and how likely they are to be connected. This circular map also explains how metabolic pathways are organized into groups, how these groups work together, and which pathways are most closely linked.

The study suggests that there are hidden geometric constraints underlying the structure of metabolic networks, despite their apparent complexity. The network topology seems to follow from simple rules based on distances in this underlying space. This means that the variety and complexity of metabolic systems may have been shaped not only by natural selection but also by the underlying geometric patterns that limit the possible paths and outcomes of evolution.
In this way, the Template Hypothesis provides a bridge between the prebiotic world and the emergence of life. As self-replicating systems become more complex and adapted through the process of natural selection, they eventually gain the ability to function independently of the fullerene template. This transition marks the beginning of life as we know it, with self-sustaining, self-replicating entities that can undergo evolution through natural selection.
To be sure, TTH isn't saying anything especially groundbreaking in this respect. There are several precedents in which early biological organisms appear to have adopted properties or benefited from interactions with inorganic molecules or minerals. Just as iron-sulfur clusters or silica provided early organisms with enhanced capabilities, fullerenes could have offered catalytic and structural advantages to nascent biological systems. The unique properties of fullerenes, such as their stability, electrical conductivity, and surface area, could have been acquired by early biomolecules to improve their functions, potentially leading to the evolution of complex structures (everything from ribosomes to microtubules).

